![]() | ||
Polar auxin transport (PAT) is the directional cell-to-cell transport of the plant hormone auxin. It results from the polar/asymmetric localisation at the plasma membrane of the auxin efflux carriers of the PIN family, which are secondary transporters that export auxin out of the cells. Polar auxin transport is one of the main processes determining the spatial distribution of auxin in plants. As such, it plays a central role in the control of plant growth and development and is tightly regulated.
Contents
- Vln2 regulates plant architecture by affecting microfilament dynamics and polar auxin transport
- Chemiosmotic model
- Acid trap
- Polarity of auxin export
- Polar auxin transport at the tissue and organ scale
- Role in plant growth and development
- In organogenesis
- In tropisms
- Generation of morphogenetic gradients
- Self organisation of polar auxin transport
- Phosphorylation of the PIN proteins
- Mechanical stress
- Vesicle Trafficking
- Inhibitors of the transport
- References
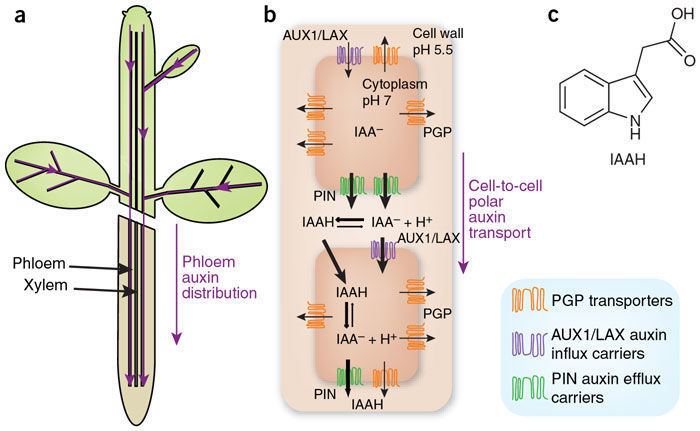
Vln2 regulates plant architecture by affecting microfilament dynamics and polar auxin transport
Chemiosmotic model
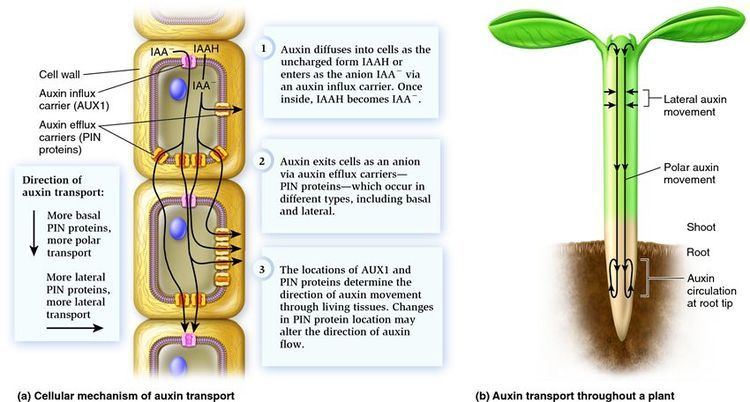
Polar auxin transport (PAT) is the directional flow of auxin molecules through the plant tissues which results from coordinated directional cell-to-cell transport.
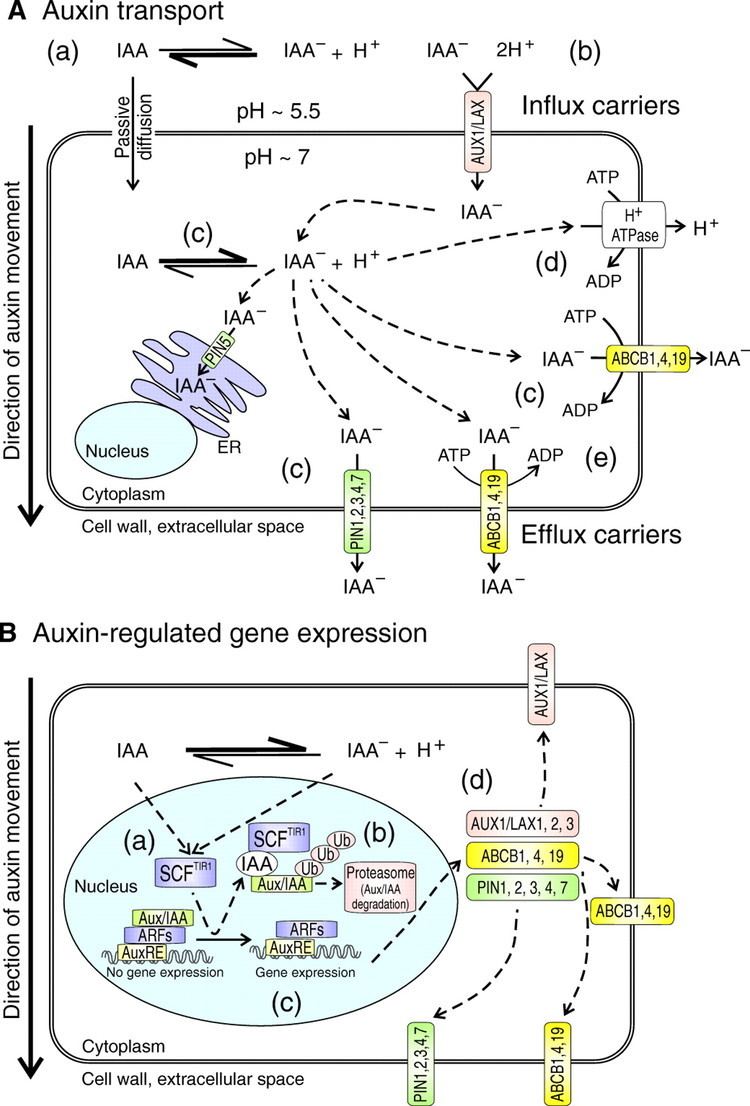
The mechanism responsible for polar auxin transport was first proposed in the seventies by Ruberry and Sheldrake and this visionary prediction was finally proven in the 21st century. The proposed mechanism, often referred as to the chemiosmotic model, entirely relies on the properties of auxin as a weak acid, and the fact that the apoplastic pH is acidic while the cytosolic pH is neutral.
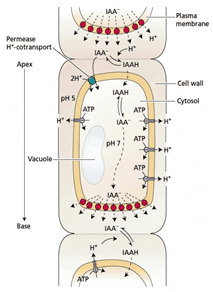
According to the chemioosmotic model, PAT results from the combination of three processes 1) The apolar influx of auxin into the cells by passive diffusion of the acidic form of auxin across the membrane; 2) The dissociation and subsequent trapping of auxin under its anionic form in the cell; and 3) the polar efflux of the anionic form of auxin through the activity of polarised auxin efflux carriers.
Acid trap
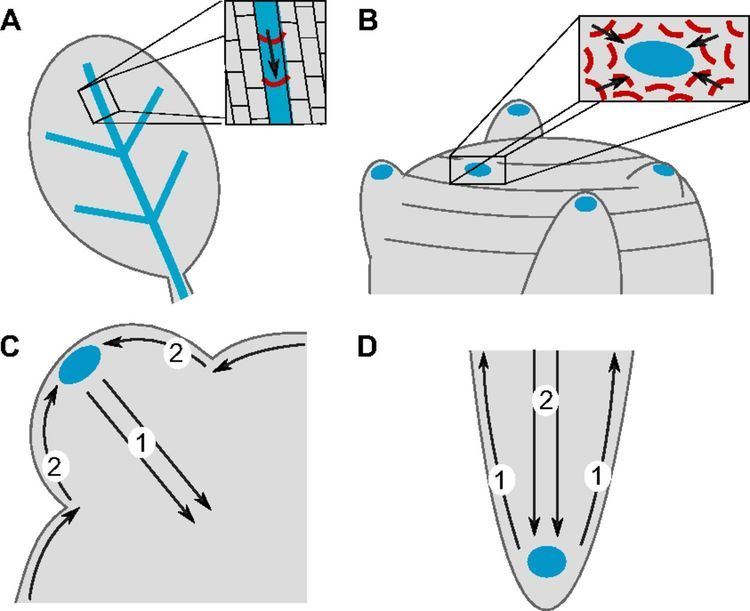
As weak acids, the protonation state of auxins is dictated by the pH of the environment; a strongly acidic environment inhibits the forward reaction (dissociation), whereas an alkaline environment strongly favors it (see Henderson-Hasselbach equation):
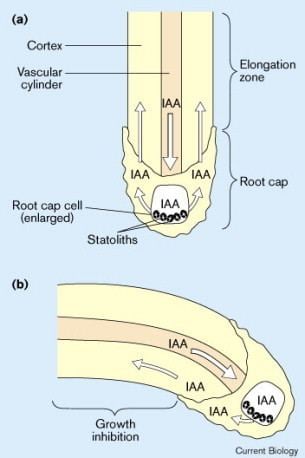
The export of auxins from cells is termed auxin efflux and the entry of auxin in to cells is called auxin influx. The first step in polar transport is auxin influx. Auxin enters plant cells by two methods, first by passive diffusion as non-ionized IAA molecule or the protonated form as IAAH across the phospholipid bilayer, or second by active co-transport in the anionic form IAA−. As IAAH is lipophilic, it can easily cross the lipid bilayer.
The inside of cells (pH ~ 7) is less acidic than the outside (the apoplast; pH ~ 5.5). So outside the cell, significant portion (17%) of the IAA molecules remain un-dissociated (proton-associated). This portion of auxin molecules is charge-neutral and therefore it is able to diffuse through the lipophilic lipid bilayer (lipid bilayer being constituent of cell membrane) into the cells. Once through the bilayer in the cell, the molecules are now exposed to the more basic pH of the cell interior, and there they dissociate almost completely, to give anionic IAA−, which being chemically polar are therefore unable to cross the lipid bilayer back again. So the auxin molecules are trapped inside the cell.
Polarity of auxin export
Once inside the cell, auxin cannot leave the cell on its own by crossing the lipid bilayer. Hence the export of auxin from the cell requires an active component in plasma membrane - i.e. some membrane transport protein. Two protein families: The PIN proteins and ABCB (PGP proteins) transporters are functioning as "auxin efflux carriers" and transport the anionic form of auxin out of the cell. While the PGP auxin efflux carriers are evenly distributed on the plasma membrane, the PIN proteins are most often maintaining polar (i.e. asymmetric) localisation on the plasma membrane (i.e. they locate mostly on one side of the cell). As a result, the PIN proteins generate a directional flow of auxin at the cellular scale.
Polar auxin transport at the tissue and organ scale
The asymmetrical cellular localisation of the PIN proteins is usually coordinated between neighbouring cells. As a consequence, a directional flow of auxin is generated at the tissue and organ scale. For instance, the cells located in the vascular tissues of the root accumulate PIN1 proteins on their basal membrane only (i.e. on their lower side). Hence, in the root vasculature, auxin is transported directionally downwards, towards the root tip.
Role in plant growth and development
Altering polar auxin transport by genetic or chemical means disrupts many developmental and growth-related processes in plants such as embryogenesis, lateral organ formation in the shoot and the roots, or root and shoot tropism.
In organogenesis
The role of polar auxin transport in organogenesis is strikingly illustrated by the phenotype of the Arabidopsis thaliana pin1 loss-of-function mutants, which lack a functional PIN1 protein. These plants are simply unable to make flowers and produce a "pin-shaped" inflorescence consisting only of a naked stem (Hence the name of the PIN gene family). Blocking auxin polar transport using the auxin transport inhibitor 1-N-Naphthylphthalamic acid (NPA) leads to a similar phenotype. Together, this indicates that PIN-mediated auxin polar transport plays a central role in flower initiation at the shoot apical meristem. Further work on this system has shown that the formation of flowers is triggered by PIN1-mediated local accumulations of auxin on the surface of the shoot apical meristem in the so-called L1 cell layer.
Mutations in the PIN genes or NPA application disrupt many other histogenetic and organogenetic processes during embryogenesis and post-embryonic development. This includes lateral root initiation, lateral root primordia patterning (morphogenetic effects), ovule formation, root hair elongation, leaf epidermis pavement cells morphogenesis, leaf margin indentation and leaf vein formation. Overall, auxin polar transport plays a central role in the spatio-temporal control of organ initiation and in tissue patterning in plants.
In tropisms
Many external and internal signals (e.g. blue light, mechanical stress, gravity or cytokinins) can interfere with PIN protein polarity and therefore with the directionality of auxin polar transport. Because auxin controls cell division and cell elongation, the change of PIN proteins localisation, and the subsequent change in auxin distribution, often lead to a change in the growth pattern.
For instance, the regulation of polar auxin transport is central in a process such as gravitropism. This process, which ensures that the root grows downwards, relies on the active redistribution of auxin by the statocytes of the columella. These cells have the ability to sense gravity thanks to special organites called the statoliths. They redistribute side-wards, to the root epidermis and the lateral root cap, the auxin that has travelled all the way down from the shoot in the central vasculature. The epidermis and lateral root cap express the PIN2 auxin efflux carrier on their apical membrane, and so transport auxin shoot-wards to the elongation zone where it locally negatively regulates cell elongation. Importantly, the columella cells always set their PIN proteins (PIN3 and PIN4) predominantly to their basal and lateral membranes, the location of which is determined by the position of statoliths within the cells: In other words, the PIN3 and PIN4 proteins always accumulate on the lower membranes of the statocytes. As a result, when the root is not vertical, the PIN distribution in the statocytes is not symmetric with respect to the longitudinal axis of the root, and more auxin is sent to the lower side and less to the upper side. The resulting higher auxin level in the lower side in the elongation zone inhibits cell elongation on that side, which causes the root tip to bend and re-orient downwards untils it becomes parallel to the gravity vector.
Similar mechanisms are working in other tropic responses, such as phototropism. The mechanisms were first described by the Cholodny-Went model, proposed in the 1920s by N. Cholodny and Frits Warmolt Went.
Generation of morphogenetic gradients
The polar auxin transport is required for generation of pattern of auxin gradients throughout the plant body. Those gradients have development significances akin to the gradients of morphogens in animal bodies. They are necessary for development, growth and response of any plant organ (such as cotyledons, leaves, roots, flowers or fruits) and response of plant to environmental stimuli known as tropisms.
Self-organisation of polar auxin transport
See also "Uneven distribution of auxin" and "Organization of the plant" in the main Auxin articleAuxin plays a central role in PIN protein polarity establishment. The regulation of PIN localisation by auxin creates a feed-back loop where PIN proteins control the directionality of auxin fluxes, and auxin in turn controls PIN proteins localisation. These interactions between auxin and its own transporters confer to the system self-organizing properties, which explains for instance phyllotaxis (the regular and geometrical arrangement of lateral organ along the stem), the formation of leaf serrations, and the formation of vascular strands.
Several mathematical models making different assumptions on the way auxin influences PIN localisation explain different observations. Some models assume PIN proteins polarize towards the neighbouring cell containing the highest cytosolic auxin concentration. These models are called "up-the-gradient" models and explain for instance phyllotaxis. Other models assume that PIN proteins (re-)localise on the side of the cell where the efflux of auxin is the highest. These models are called "with-the-flux" models and rather explain the formation of vascular strands in leaves.
The molecular mechanism responsible for these different behaviours of the system (with-the-flux and up-the-gradient) is not yet fully understood and is still subject to many controversies. Noticeably, an auxin receptor protein called ABP1 is thought to play a potentially significant role in the control of PIN proteins polarity by auxin.
Phosphorylation of the PIN proteins
The intensity and direction of polar auxin transport can be regulated by the reversible phosphorylation of PIN proteins. Two families of protein kinases can phosphorylate the PIN proteins: the AGC kinases and the D6PK kinases. The regulation of PIN proteins by those kinases is essential in many developmental processes.
The AGC kinases, such as PINOID (PID), phosphorylate the PIN proteins at several sites located on their large intracellular hydrophylic loop. The phosphorylation status at those particular residues control the subcellular localisation of the PIN proteins. In root cells for instance, non-phosphorylated PINs locate on the basal/lower side of the cells while phosphorylated PIN proteins locate on the apical/upper side of the cells.
The D6 protein kinases (D6PK) phosphorylate PIN proteins on different residues than the AGC kinases and by doing so, they increase the ability of the PIN proteins to transport auxin i.e. their molecular permeability to auxin.
Mechanical stress
Mechanical signals have been proposed to regulate PIN polarity.
Vesicle Trafficking
The asymmetrical localisation of PIN efflux carrier protein at the plasma membrane has been shown to involve the localized targeting of vesicles and the local regulation of clathrin-mediated endocytosis. The latter involves the actin cytoskeleton.
Inhibitors of the transport
In research, 1-N-Naphthylphthalamic acid (NPA) and 2,3,5-triiodobenzoic acid (TIBA) are used as specific inhibitors of the auxin efflux.
Quercetin (a flavonol) and Genistein are naturally occurring auxin transport inhibitors.
9-Hydroxyfluorene-9-carboxylic acid (HFCA), TIBA, and trans-cinnamic acid (TCA) are also example of Polar Auxin Transport Inhibitors. They prevent the development of the bilateral growth of the plant embryo during the globular stage. All 3 inhibitors induce the formation of fused cotyledons in globular but not heart-shaped embryo.